
La stratification thermique des lacs détermine l’emplacement des poissons, mais modifie aussi la capacité de la télémétrie acoustique à les détecter.
– Par Mathew Wells, Yulong Kuai, and Yulu Shi –
L’utilisation de l’habitat des poissons dans les grands lacs et dans l’océan côtier est en partie déterminée par la stratification thermique, les poissons choisissant des régions qui correspondent à leurs préférences thermiques. La stratification de la densité sous-entendue par une stratification d’eau chaude au-dessus d’eau froide contrôle également les profils d’oxygène dissous et la localisation du plancton et des espèces proie, qui déterminent aussi l’utilisation de l’habitat par les poissons. Cependant, cette stratification n’est pas stable, et la thermocline d’un grand lac est toujours en mouvement constant en raison du forçage du vent. Cela signifie que les poissons doivent également ajuster constamment la profondeur pour rester dans leurs préférences thermiques. Les scientifiques emploient une série de technologies de détection acoustique pour surveiller l’utilisation de l’habitat par les poissons. La vitesse de propagation du son dans l’eau est principalement une fonction de la densité, et dans les lacs d’eau douce, la densité est principalement une fonction de la température, donc les gradients de vitesse du son à travers les thermoclines peuvent potentiellement focaliser ou défocaliser les signaux sonores et changer l’efficacité de la détection des poissons. Ainsi, si la thermocline change rapidement de profondeur dans un système aquatique, les poissons sont susceptibles de changer de position en même temps que l’efficacité de leur détection change. Cela complique notre compréhension de l’utilisation de l’habitat des poissons dans les systèmes à thermoclines dynamiques
The habitat usage of fish in large lakes and the coastal ocean is in part determined by thermal stratification, whereby fish choose regions that match their thermal preferences. The density stratification implied by a stratification of warm water above cold water also controls the dissolved oxygen profiles and the location of plankton and prey species, which also determine fish habitat usage.
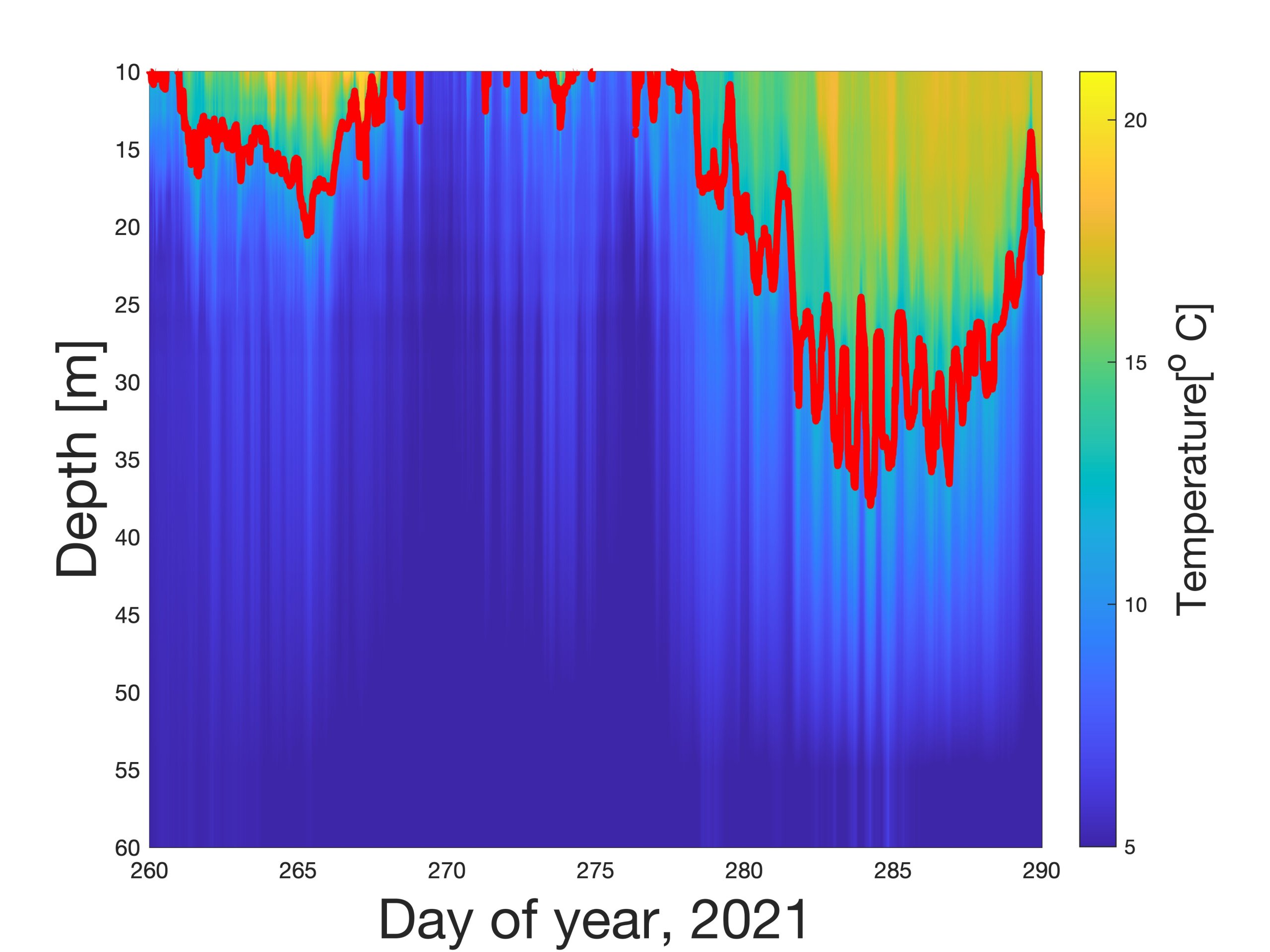
However, this layering is not stable, and the thermocline of a large lake is always in constant motion due to wind forcing. This means fish must also constantly adjust depth to stay within their thermal preferences. Scientists use a range of acoustic detection technology to monitor fish habitat usage. The speed of sound propagation in water is mainly a function of density, and in freshwater lakes, density is mainly a function of temperature, so the speed of sound gradients across thermoclines can potentially focus or defocus sound signals and change how efficiently fish can be detected. Thus, if the thermocline is rapidly changing depth in an aquatic system, fish are likely to be changing positions at the same time as the efficiency of detecting them changes. This complicates our understanding of fish habitat usage in systems with dynamic thermoclines.
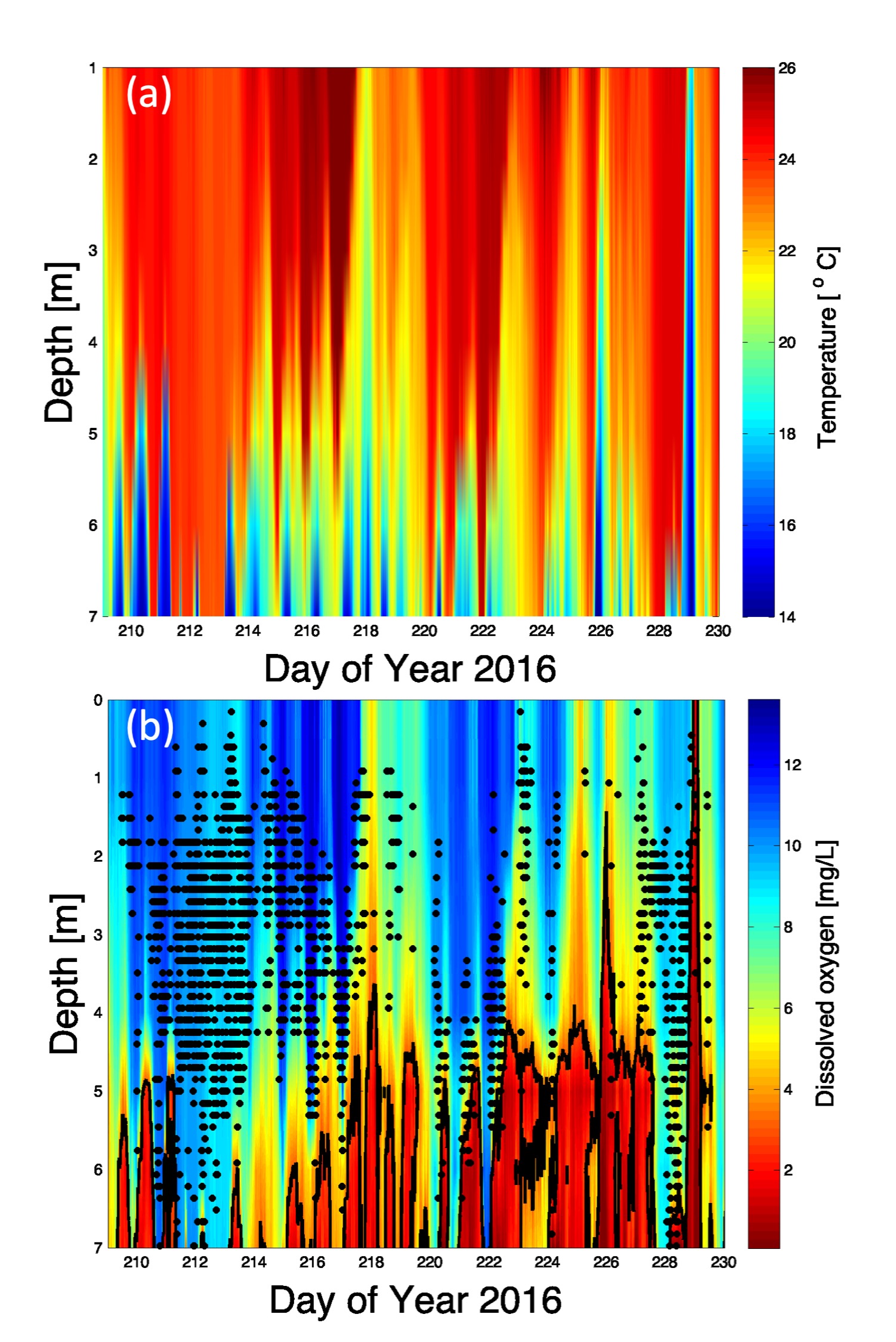
In Figure 1 we show thermal data we collected in Lake Ontario near Toronto in early summer 2021. The surface of the lakes was as warm as 22oC, while the deepest waters were near 5oC. The red line is the 12oC isotherm and its depth can move over 20 m a day, which would influence the depth usage of cold water fish like trout or cisco. There is evidence for both 17 hour Poincare waves and 1-3 day Kelvin waves driving the large thermocline movements in Lake Ontario. The amplitude of such internal waves is greatest in large lakes which have a long fetch. In large lakes, such as the 50 km wide Lake Simcoe, these waves can be modified by Coriolis forces (Flood et al 2020). Even in smaller lakes, such as the 7 km long Hamilton Harbour, diurnal winds can lead to vertical movements of the thermocline of 5-10 m (Flood et al. 2021a).
When a fish’s preferred temperature range coincides with the thermocline, the fish responds to internal waves by changing depth (Flood et al. 2021b). For instance, in Lake Simcoe, cold water Cisco stayed in colder deeper waters and tracked the moving 12oC isotherm (Flood et al. 2021b). Thermoclines in productive lakes often coincide with oxyclines, as the thermocline represents a barrier to mixing and supply of oxygen to deeper waters. In lakes with anoxic conditions at depth, fish may avoid the low oxygen zones and only use warm oxygenated water above the thermocline. Such behaviour was seen in Bass in Hamilton Harbour, where winds drove a 12 hour internal seiche that lead to extreme variability in dissolved oxygen in the littoral regions of the 7 km long harbour (Brooks et al. 2021). These fish were observed to adjust their position in response to the variable thermocline depth to stay above the thermocline in the warmer oxygenated waters (Figure 2).
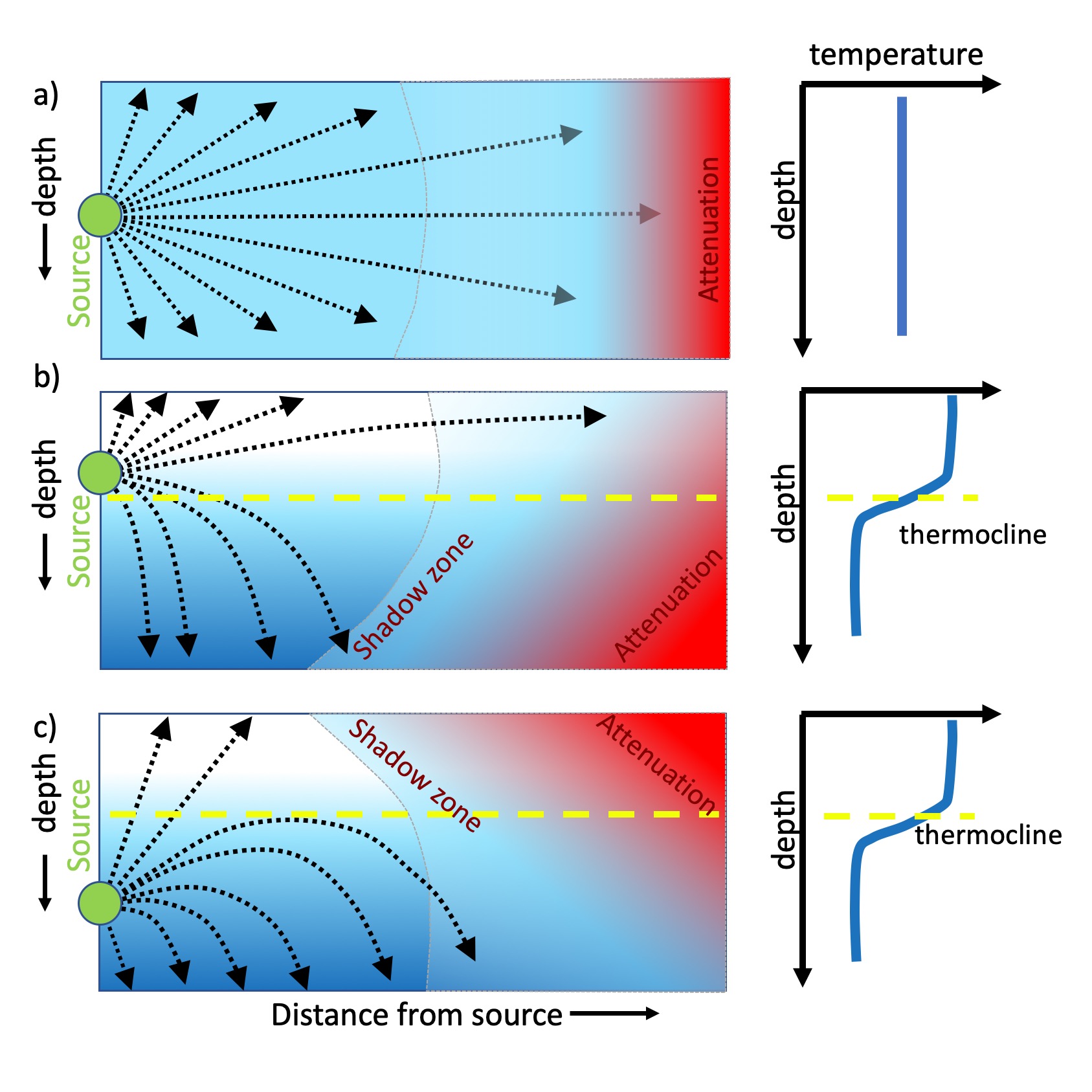
A recent technical revolution in fisheries research is the use of acoustic tags to directly track fish positions. A small tag is surgically implanted in a representative sample of the fish population and their location is then monitored through an array of bottom-mounted receivers. The presence of thermal stratification directly influences where fish will be in the water column, and thermal stratification also impacts how the acoustic transmissions propagate. In particular, the associated speed of sound gradients induced by temperature gradients have implications for the detection efficiency of acoustic telemetry devices and detection range. Detection efficiency can be influenced by thermal stratification, because of that, sound waves from an acoustic tag can refract and bend as they move from the warmer waters to a receiver on the lake bed, where water is usually cooler. Thus it leads to greater divergence of acoustic signals, and hence causes more sound attenuation that influences the overall performance of fish monitoring using acoustic tags. Depending on how pronounced the speed of sound gradients are, and the relative vertical locations of tagged fish and receivers, detection range may be significantly impacted in thermally stratified systems (Figure 3). According to the study carried out by Kuai et al. (2021), found that the steepest speed of sound gradient could reach 10.38 m/s m-1 across the thermocline of Lake Ontario, which led to the speed of sound difference between top and bottom of the water column reaching 60 m/s. The detection range of various Vemco transmitters could reach above 650 m when the water was isothermal, and it decreased to 350 – 450 m, when the water was stratified and the transmitters and receivers were situated at different sides of thermocline (Kuai et al. 2021). Thus, understanding the influence of thermal stratification to designing acoustic telemetry studies can increase the detection efficiency and detection range, and hence, improving the performance of acoustic tags.
One prominent example of a major fisheries telemetry project is GLATOS, the Great Lakes Acoustic Telemetry Observation System (Krueger et al. 2018), which now has placed acoustic tags in over 10,000 fish, with their locations recorded at over 1000 receiver stations, resulting in over 300 million position reports. Going forward, in Lake Ontario alone there are >100 bottom receivers, 300-500 active transmitters (fish), and 5-10 million potential detections over the next 3 years. Both transmitters and receivers usually record temperature as well, representing an untapped physical record of water temperatures. The strong seasonal thermal stratification in the Great Lakes represents some of the steepest sound speed gradients in any aquatic system. So far the influence of thermal stratification variability has not been considered as part of the acoustic detection array design, nor has the role of variability of thermal stratification been considered in driving some of the variability in fish habitat usage, even though we know different parts of Lake Ontario have strikingly different variability in thermocline depth (Stewart and Robertson, 1990). In order to understand how internal waves might structure the location of fish in lakes, it is first important to understand how variable thermocline movements are around the Great Lakes. Key questions are 1) What locations around the perimeter of large lakes have the largest internal waves? 2) Can the distributed receiver network give extra insight into internal wave dynamics in the Great Lakes? 3) How can we best mine the big data of GLATOS fish telemetry records to correlate fish movements with thermocline motions?
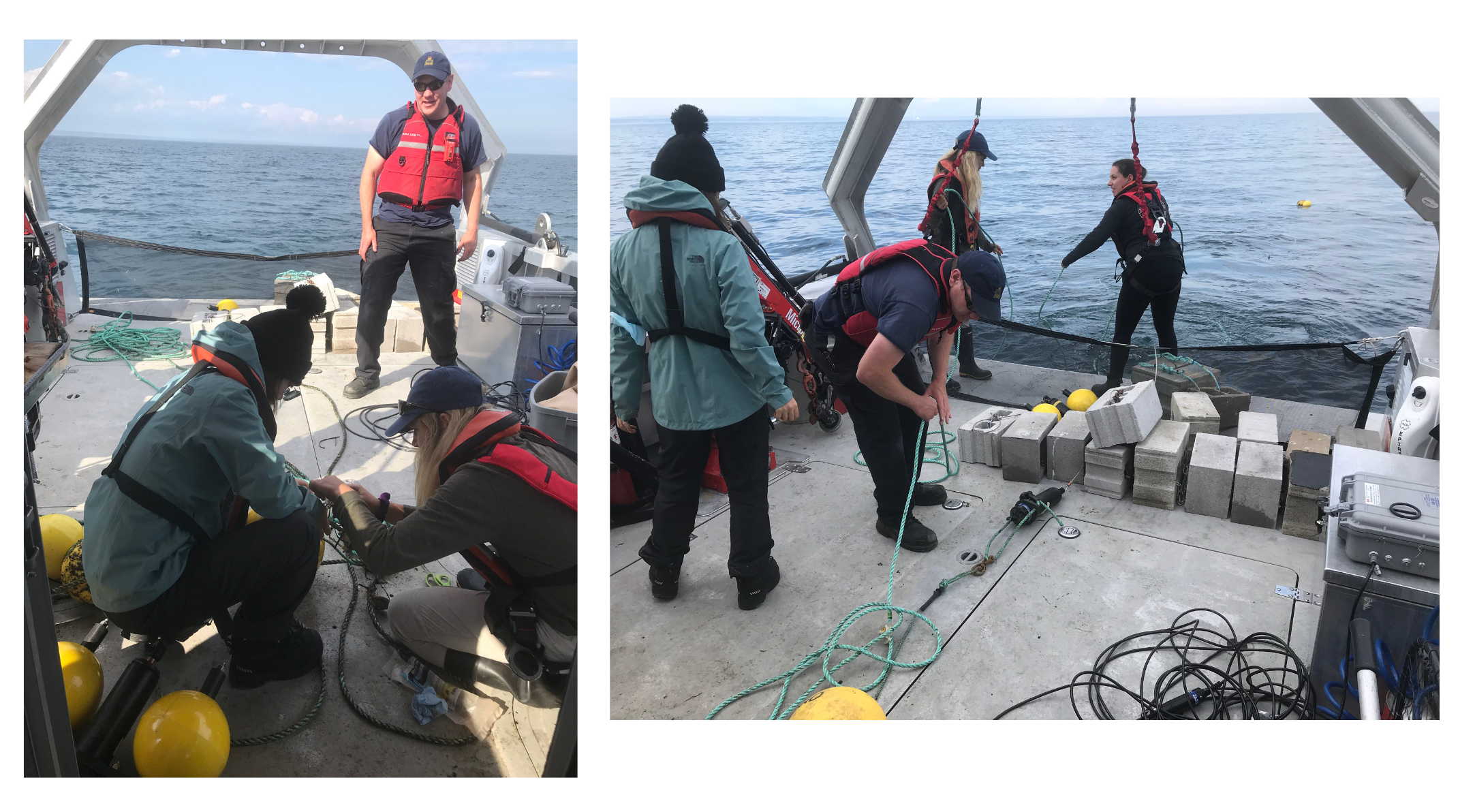
Dr. Mathew Wells is a Professor in the Department of Physical and Environmental Sciences at the University of Toronto Scarborough. His Environmental Fluid Dynamics group studies flows in lakes, rivers and the coastal ocean, in order to understand the transport and distribution of biology, sediment and chemicals.
Yulong Kuai is a PhD student in the Environmental Science program at the University of Toronto. For his Masters degree he studied quantifying the potential impacts of temperature and dissolved oxygen variability on fish habitat during the summer stratified period in Hamilton Harbour, Ontario. His current doctoral research on western Lake Erie investigates the correlation among physical drivers that triggers hypoxia and potential algal bloom in this large polymictic basin.
Yulu Shi is a fourth year undergraduate in the Specialist co-op program in Geoscience at the University of Toronto Scarborough. For her fourth year research project she will quantify the thermal stratification patterns across Lake Ontario and the dynamics of the thermocline depth as a function of wind patterns and bathymetry.
References
- Brooks, J.L., Midwood, J.D., Smith, A., Cooke, S.J., Flood, B., Boston, C.M., Semecsen, P., Doka, S.E., Wells, M.G., (2022). Internal seiches as drivers of fish depth use in lakes. Limnology and Oceanography. 67 1040-1051
- Flood, B., Wells, M., Midwood, J. D., Brooks, J., Kuai, Y., Li, J. (2021). Intense variability of dissolved oxygen and temperature in the internal swash zone of Hamilton Harbour, Lake Ontario. Inland Waters, 11(2), 162-179.
- Flood, B., Wells, M., E Dunlop, J Young (2021) Vertical oscillations of the thermocline caused by internal waves modify coldwater pelagic fish distribution: results from a large stratified lake. Journal of Great Lakes Research 47 (5), 1386-1399
- Flood, B., Wells, M.G., E Dunlop, J Young (2019) Internal waves pump waters in and out of a deep coastal embayment of a large lake. Limnology and Oceanography 65 (2), 205-223
- Krueger, C. C., Holbrook, C. M., Binder, T. R., Vandergoot, C. S., Hayden, T. A., Hondorp, D. W., Nate, N., Paige, K., Riley, S.C., Fisk, A.T., Cooke, S. J. (2018). Acoustic telemetry observation systems: challenges encountered and overcome in the Laurentian Great Lakes. Canadian Journal of Fisheries and Aquatic Sciences, 75(10), 1755-1763.
- Kuai, Y, NV. Klinard, A T. Fisk, T B. Johnson, E A. Halfyard, D M. Webber, S J. Smedbol, M G. Wells. (2021). Strong thermal stratification reduces detection efficiency and range of acoustic telemetry in a large freshwater lake. Animal Biotelemetry 9, 1-13.
- Stewart, T.J., Robertson, D. G. (1991). Lake Ontario temperature studies. Ontario Ministry of Natural Resources, Lake Ontario Fisheries Unit 1990 Annual Report, LOA, 91(20), 1-5.
- Wells, M. G., Li, J., Flood, B., Kuai, Y., Brooks, J. L., Cooke, S. J., Semcesen, P., Midwood, J. D. (2021). Speed of sound gradients due to summer thermal stratification can reduce the detection range of acoustic fish tags: results from a field study in Hamilton Harbour, Ontario. Canadian Journal of Fisheries and Aquatic Sciences, 78(3), 269-285.
lac ontario, lacs, mathew wells, poisson, yulong kuai, yulu shi